Deciphering the Science: Understanding the Mechanisms and Benefits of Soil Organic Carbon in Croplands
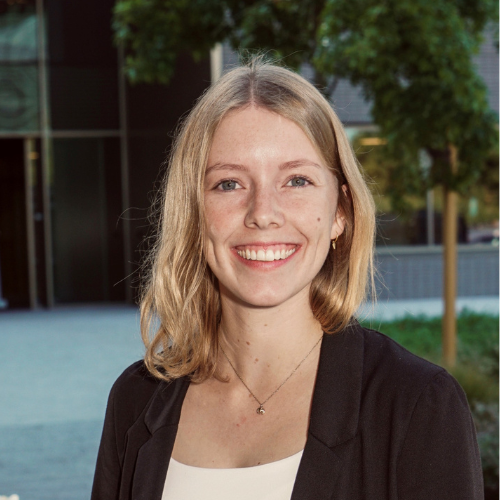
Hannah Ovelhey studied agricultural sciences, specialising in agricultural economics, in Bonn, Göttingen and Milan. She then worked for some time in the Netherlands as a project manager for a seed company. After her time at NEXAT as an agricultural economist, she is now doing her doctorate in horticulture at Osnabrück University of Applied Sciences on the topics of biofortification and greenhouse gas emissions in broccoli cultivation.
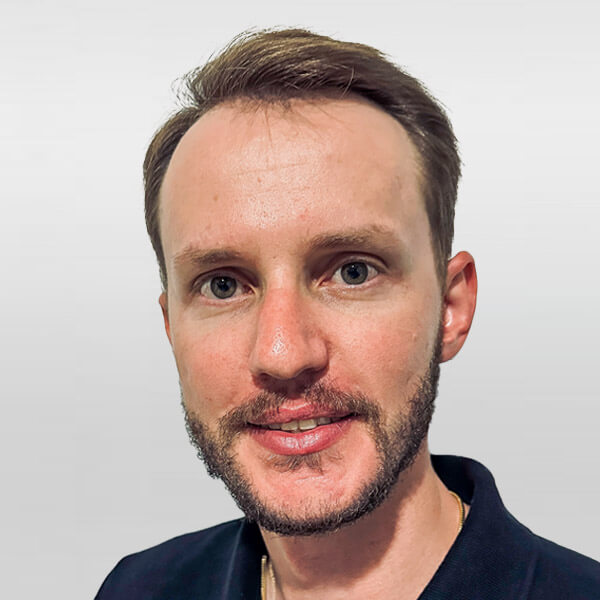
Vinicius Marchioro, agricultural engineer and doctor of agricultural sciences, is the country manager for NEXAT in Brazil. Specialising in chemical and biological soil management, conservation soil management and restoration of degraded land and soil water, he is an absolute soil expert who shares his expertise in this article.
Heutzutage wird viel über die Erhöhung der organischen Substanz (Humus oder SOM) im Boden gesprochen, als wirksame Maßnahme um dem Klimawandel entgegenzuwirken und gleichzeitig Geld mit dem Verkauf von CO2 Zertifikaten zu verdienen. This article explains the natural processes underlying humus, illustrates the mechanisms of humus formation and highlights both the benefits and limitations.
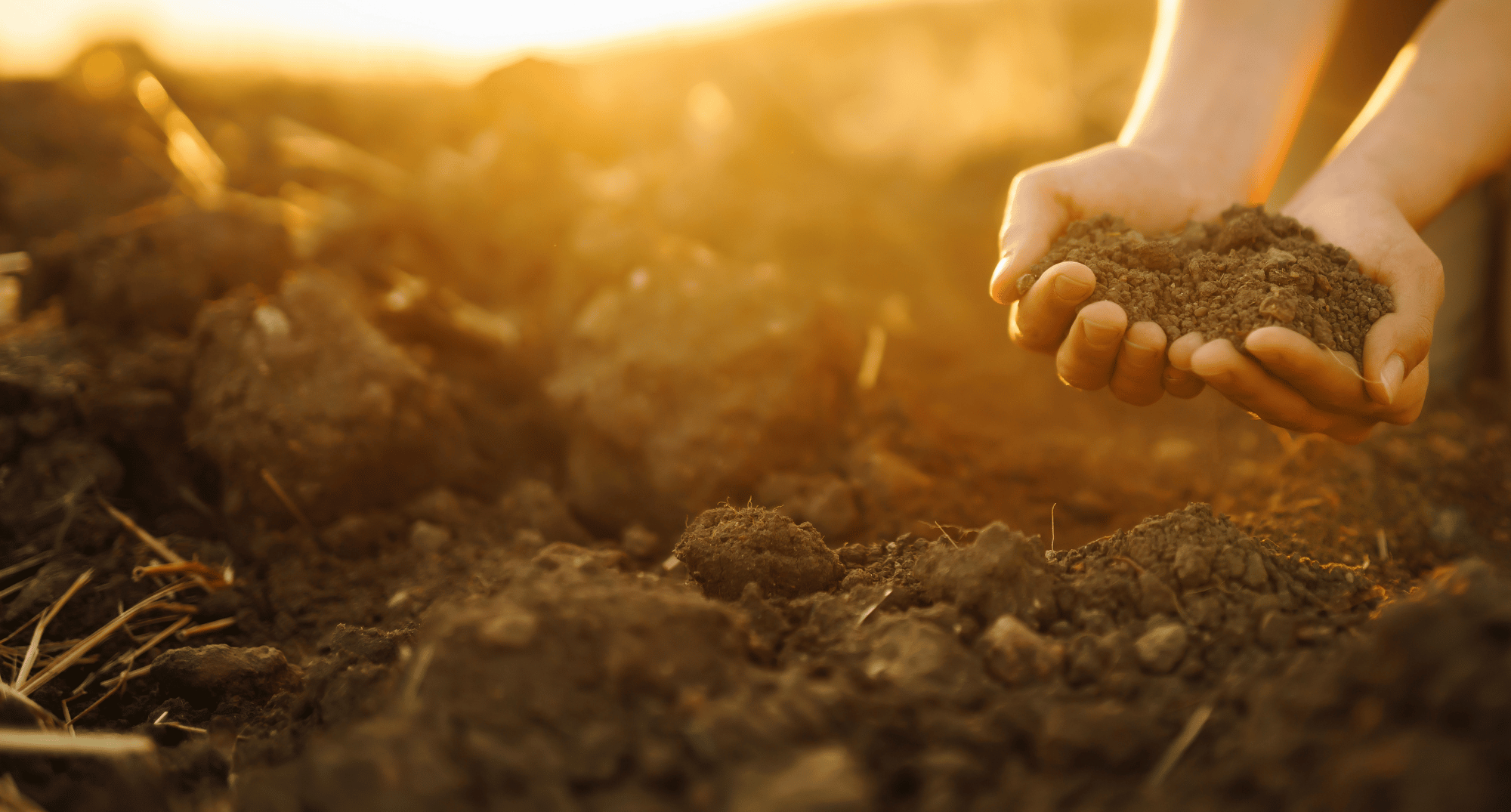
What is Soil Organic Carbon?
Soil carbon is the carbon atoms stored in the soil in various solid forms. One can further classify:
- Soil Organic Carbon (SOC): Measure of the organically bound carbon (C) contained in the soil.
- Soil Inorganic Carbon (SIC): measure of minerally bound carbon (C) contained in the soil.
- Organische Substanz (OS, Humus): sich allmählich zersetzende organische Verbindungen pflanzlichen, tierischen und mikrobiellen Ursprungs; Verbindungen bestehen aus C, O, H, N, S, P1; der C-Anteil beträgt 50-58%.
SOC can be seen as in a continuous state of turnover, it is mineralized, released and replaced by new carbon inputs. It is relevant to understand that SOM is not one single substance, but a gradient of different organic compounds that vary with regards to e.g. composition, energy content or likeliness to bind with other materials. Kohlenstoff ist in dieser organischen Substanz in verschiedenen physikalischen oder chemischen Formen gebunden, und dementsprechend kann die Zersetzung schneller (1-2 Jahre) oder langsamer (> 1.000 Jahre) erfolgen. ). Especially the faster cycling pools (fast decomposition) are associated with crop yield because SOM decomposition releases nutrients that become available for plant growth (as mentioned SOM contains various nutrients, so when it is decomposed all of these become available). Slower cycling pools are more relevant for long-term carbon storage in the soil and mitigating climate change.
There are other approaches of separating SOC carbon into different groups (e.g. humic acids/fulvic acids; stabile/labile pool) however, these are more conceptual than measurable and are rather controversial in the scientific world2.
carbon cycle
As mentioned, SOC is a continuum – a dynamic process involving continuous carbon inputs and outputs. Carbon enters the soil through plant photosynthesis, primarily via rhizodeposition and litter decomposition. Rhizodeposition involves carbon compounds released through root exudates, mucilage, and root cell sloughing, while litter contributes carbon when plants or their parts decompose.
Microbes and fauna play a crucial role in decomposing litter and exudates and recycling carbon into various forms. Within these processes nutrients are released, which are then utilized again by microbes and fauna, enhancing soil fertility, but are also available for plant uptake. SOM can thus be seen as a transient storage for nutrients.
During these microbial decomposition processes, termed microbial respiration, microbes release carbon back into the atmosphere, primarily as CO2 under aerobic conditions and as CH4 under anaerobic conditions. SOC can also be lost through leaching, erosion, and harvesting of plant parts. Understanding these processes is essential for managing SOC levels and optimizing soil health and ecosystem function.
Important to remember:
- Der Bodenkohlenstoff wird erhöht, wenn Anreicherung > Zersetzung und Auswaschung.
- The balance of C inputs and C outputs determine potential of sequestering C in the soil.
- SOC is lost more quickly than it can be gained.
Factors influencing carbon sequestration decomposition
During the decomposition process of organic matter, the carbon is built into different compounds, some of which are less prone to decomposition/less accessible to organisms and can thus stay in the soil for millennia. There are different forms of stabilization, such as physical aggregation (e.g. fine roots, clay and microbial residues build clumps), chemical bonds (SOC binds to mineral soil particles), biochemical bonds (e.g. compounds such as waxes or lipids)3.
There are different factors influencing the magnitude of stabilization as well as the processes of sequestration decomposition.
1. Soil type
The soil type significantly impacts SOC content through various factors, including texture. Finer-textured soils tend to have higher SOC content due to increased surface area for chemical binding of SOM to clay particles, thereby enhancing stabilization. Consequently, under similar climatic conditions, fine-textured soils typically exhibit higher SOM levels compared to coarse-textured soils.
Additionally, soil depth plays a crucial role in SOC storage, with deeper soil profiles containing in total rather more SOM until reaching parent material, simply because there is more soil. Bulk density and aeration also influence SOM dynamics. Soils with lower aeration, such as those found in wetlands, experience slower decomposition of SOM due to reduced microbial activity, thereby affecting SOC accumulation rates.
2. Climate
Apart from soil texture, climate also influences SOC dynamics, mainly in form of moisture and temperature. Colder and wetter climates tend to favor the buildup of organic carbon in soil. These conditions slow down the decomposition process, allowing for greater accumulation of organic matter over time. Conversely, under warmer climates, soil microbes are typically more active, leading to increased decomposition rates of organic matter. This heightened microbial activity accelerates the breakdown of organic carbon and reduces its retention in the soil.
It is crucial to understand that an activity that builds carbon on one soil type and climate may be ineffective on another.
3. Management practices
Introducing additional crops, such as cover crops, increases biomass production and adds organic matter to the soil. Conversely, leaving soil bare results in minimal carbon input from plant growth, leading to a decline in SOC over time. Fertilization is another strategy employed to enhance crop productivity, consequently increasing biomass and carbon input into the soil. Organic fertilization methods further contribute to SOC by returning carbon-rich materials to the soil. Retaining crop residues on the field, rather than removing them, helps maintain soil organic matter levels. This practice ensures a continuous supply of organic material for microbial decomposition and SOC accumulation.
Recent studies have highlighted the importance of living roots in carbon input to soils. While aboveground inputs in the form of residues or fertilizer are significant, living roots play a critical role in continuously supplying carbon to the soil through root exudation and turnover processes. Understanding and implementing these practices are essential for promoting soil health and enhancing carbon sequestration in agricultural systems4.
Drainage and irrigation practices play a key role in regulating soil water content, which, in turn, affects the activity of microorganisms involved in decomposition. Optimal water levels are essential for maintaining microbial activity, as both excessive moisture and water deficiency can decrease microbial function.
Tillage practices also have profound effects on SOC dynamics. When soil is tilled, mechanical disturbance disrupts soil aggregates, exposing SOC previously protected within aggregates and accelerating decomposition processes. Additionally, tillage can increase SOC losses through erosion. Furthermore, tillage influences soil temperature, with tilled soils typically warming up faster than untilled soils. This temperature increase enhances microbial activity, further accelerating decomposition rates.
However, the effects of tillage on SOC dynamics are contingent upon soil structure and climatic conditions. Studies have shown that SOC losses due to tillage are exacerbated in regions with higher precipitation levels. Conversely, the benefits of no-tillage practices are more pronounced in humid or sub-humid climates.
It is important to note that the level of SOM does not increase indefinitely. Each management adjustment to increase SOC will lead to a new equilibrium5.
Abbildung 1: SOM and crop rotation. From Sousa et al. (2004)
Darüber hinaus gibt es einen natürlichen Sättigungsgrad des Bodenkohlenstoff Gehalts, bei dem der Gehalt unabhängig von den Bewirtschaftungsmaßnahmen nicht weiter erhöht werden kann. Die meisten landwirtschaftlich genutzten Böden haben jedoch in der Regel einen zu geringen C-Input, um die Sättigung zu erreichen6.
Abbildung 2: Erhöhung und Sättigung des Bodenkohlenstoffs. Nach West und Six (2007)
Benefits of Soil Organic Carbon
SOC plays a pivotal role in soil dynamics, influencing various aspects of soil structure and function. Through its binding with mineral soil particles, SOC alters soil structure, leading to enhanced soil strength, reduced bulk density, improved pore size distribution, and decreased erodibility. This improved soil structure augments water holding capacity, fostering resilience to drought conditions. Additionally, the negatively charged nature of SOM enables it to sequester positive cations such as potassium, magnesium, and iron, thereby reducing leaching and enhancing nutrient availability for plant uptake.
As SOM decomposes, it releases essential nutrients, nourishing crops and enhancing nutrient holding capacity and water retention abilities. Concurrently, SOM serves as a safeguard against pollutants by retaining them within the soil, preventing their leaching into groundwater and contributing to water purification processes. Moreover, SOC influences soil pH and buffering capacity, with soils rich in SOC exhibiting greater resistance to acidification.
Furthermore, the accumulation of SOM promotes biodiversity within the soil ecosystem, fostering a thriving community of microorganisms and other living organisms. This rich biological diversity contributes to soil health and ecosystem resilience. Ultimately, the presence of ample SOM enhances soil fertility and productivity.
Zusätzlich zu den agronomischen Vorteilen bietet ein erhöhter Anteil an organischer Substanz enormes Potenzial zur Kohlenstoffanreicherung dar, wodurch der Atmosphäre effektiv CO2 entzogen wird. This process represents a low-cost option for mitigating climate change while simultaneously enhancing soil health. Comparatively, soils store significantly more carbon than plant biomass, highlighting the importance of soil carbon management in carbon cycling processes.
Abbildung 3: Verteilung des terretrischen (Boden und Vegetation) organischen Kohlenstoffs nach Klimaregion des IPCC. Nach Trivedi et al. (2018)
In many ways these two goals connect: e.g. deeper roots are crucial for increasing yield and at the same time increasing soil carbon storage. . Root development is again linked with soil structure and water availability: improved soil structure improves root growth as well as water holding capacity which in turn leads again to more carbon being stored in the soil in the form of SOM.
There is indication that carbon stabilized in aggregates stores more carbon in the soil but is not beneficial for yields9. However, in many of today’s agricultural soils, the share of SOM is so far depleted that any increase will be beneficial in terms of agronomic aspects as well as carbon storage.
Carbon Credits
This is where carbon credits come into play. Many companies try to decarbonize their supply chains and reduce their carbon footprint by reducing emissions from their operations. However, in some operations it is not possible to further reduce emissions, thus a market has developed where companies (in this case farmers) remove additional carbon from the atmosphere, e.g. via binding it in the soil in form of SOM and can then sell this in form of a credit to others, which in turn compensate their emissions via these credits instead of actually reducing their emissions. There are many providers offering farmers an application to track the built-up credits and sell them.
Despite the substantial carbon storage potential of soils, restoring SOC to its original natural levels within relevant timescales for climate mitigation remains a challenge in many cases. Nevertheless, prioritizing sustainable land management practices that promote SOC accumulation can contribute to both climate change mitigation, soil ecosystem resilience and increased productivity. The goal should be to maintain an equilibrium between carbon input and loss at a local optimum level for adaptation to local conditions.
Quellen und weiterführende Literatur:
1) A. Edward Johnston, Paul R. Poulton, and Kevin Coleman, “Soil Organic Matter: Its Importance in Sustainable Agriculture and Carbon Dioxide Fluxes,” in Advances in Agronomy, vol. 101 (Elsevier, 2009), 1–57, https://doi.org/10.1016/S0065-2113(08)00801-8.
2) Johannes Lehmann and Markus Kleber, “The Contentious Nature of Soil Organic Matter,” Nature 528, no. 7580 (December 2015): 60–68, https://doi.org/10.1038/nature16069.
3) Brajesh Kumar Singh, ed., Soil Carbon Storage: Modulators, Mechanisms and Modeling (London: Academic Press, 2018).
4) Stephen A. Wood and Mark A. Bradford, “Leveraging a New Understanding of How Belowground Food Webs Stabilize Soil Organic Matter to Promote Ecological Intensification of Agriculture,” in Soil Carbon Storage (Elsevier, 2018), 117–36, https://doi.org/10.1016/B978-0-12-812766-7.00004-4.
5) Catherine E. Stewart et al., “Soil Carbon Saturation: Concept, Evidence and Evaluation,” Biogeochemistry 86, no. 1 (September 10, 2007): 19–31, https://doi.org/10.1007/s10533-007-9140-0.
6) D. M. G. de SOUSA, Cerrado: correção do solo e adubação (Embrapa Informação Tecnológica; Planaltina, DF: Embrapa Cerrados, 2004).
7) Tristram O. West and Johan Six, “Considering the Influence of Sequestration Duration and Carbon Saturation on Estimates of Soil Carbon Capacity,” Climatic Change 80, no. 1–2 (January 16, 2007): 25–41, https://doi.org/10.1007/s10584-006-9173-8.
8) Pankaj Trivedi, Bhupinder P. Singh, and Brajesh K. Singh, “Chapter 1: Introduction, Importance, Status, Threat, and Mitigation,” in Soil Carbon Storage (Elsevier, 2018), 1–28, https://doi.org/10.1016/B978-0-12-812766-7.00001-9.
9) Anna M. Cates and Matthew D. Ruark, “Soil Aggregate and Particulate C and N under Corn Rotations: Responses to Management and Correlations with Yield,” Plant and Soil 415, no. 1–2 (June 2017): 521–33, https://doi.org/10.1007/s11104-016-3121-9.